Biocompatible PDMS-b-PMOXA Polymersomes for Cell Type Specific Drug Targeting
Introduction
Many promising therapeutic compounds suffer from disadvantages such as low bioavailability, rapid clearance, and high systemic toxicity. To overcome these challenges, nanoparticles such as polymersomes can be used as promising drug delivery systems. Polymersomes are vesicles formed by self-assembly of amphiphilic block co-polymers such as poly(dimethylsiloxane)-b-poly(2- methyloxazoline) (PDMS-b-PMOXA). PDMS-b-PMOXA is a promising block co-polymer because the individual polymer blocks have been reported to be biocompatible. Herein, we present the formulation of polymersomes based on PDMS-b-PMOXA, and we demonstrate slow drug release of incorporated model drug (i.e., carboxyfluorescein, CF) in vitro. In addition, we focus on the surface modification of PDMS-bPMOXA polymersomes (PP) for targeted drug delivery to hepatocytes. Potential toxicity of the resulting polymersomes is assessed in vitro and in vivo. 1
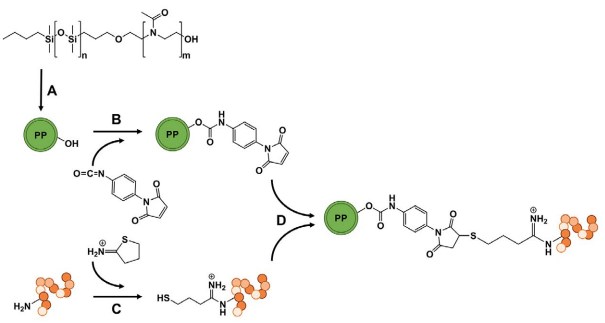
Experimental Approach and Results
PP were prepared by thin-film rehydration and subsequently modified to obtain targeted vesicles (Fig. 1). All PP variants were routinely analyzed by dynamic light scattering (DLS) for average size and size distribution (Table 1). The different modifications resulted in monodisperse suspensions of particles with hydrodynamic diameters of around 150 nm. Cryo-transmission electron microscopy (cryo-TEM) confirmed size and vesicle morphology (Fig. 2A). The polymersomes could be stored in Dulbecco’s phosphate-buffered saline (DPBS) at 4°C for at least 4 months without significant change in particle size and polydispersity index (PDI) and without loss of functionality as confirmed by cellular uptake experiments. In addition, the integrity of the particles was tested under forced stress conditions.
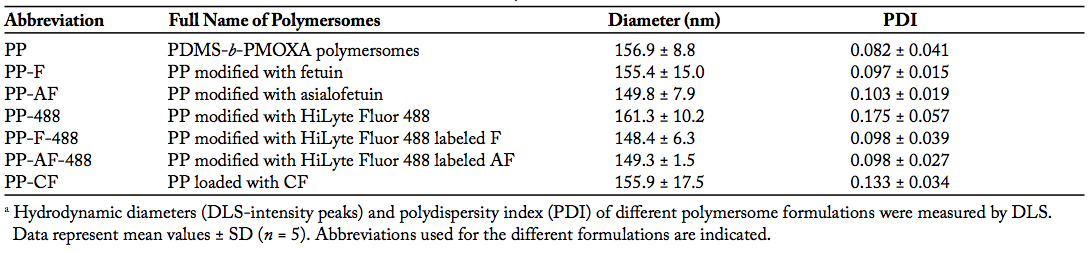
Unmodified PP were incubated in DPBS, 3% bovine serum albumin (BSA), or 50% fetal calf serum (FCS) for 7 days at 37°C. As outlined in Figure 2B, no statistically significant changes in particle diameters occurred over time. To demonstrate that PDMS-b-PMOXA forms tight vesicles, we encapsulated CF as a model drug into PP and subsequently measured CF release into DPBS at different temperatures over 96 h using a microdialysis device (Fig. 2C). CF release was temperature dependent and occurred in a slow and sustained manner over a long period of time. No initial burst release was observed at any of the tested temperatures, and no plateau was reached within the duration of the experiment. Moreover, a similar release profile was observed in buffers containing naturally occurring serum proteins (3% BSA and 50% FCS, observation period 48 h). Using the standard thin-film rehydration method, the achieved loading capacity was 1.5 ± 0.2 nmol of CF per mg of PP. This seems low. However; to achieve therapeutic effects, alteration of pharmacokinetics is the most important factor. Nanoparticles can shift the balance in off- and on-target accumulation. Therefore, already a low percentage of nanoparticles delivered to the diseased tissue may offer a benefit to the patients by reducing severe side effects of encapsulated drugs.2
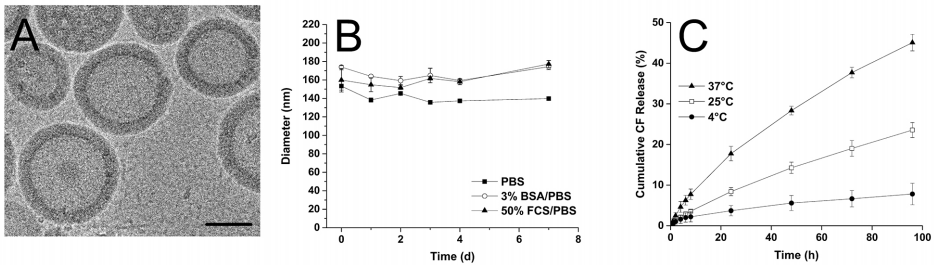
For hepatocyte-specific targeting, we selected the asialoglycoprotein receptor (ASGPR),3 and its naturally occurring ligand asialofetuin (AF) was covalently linked to PP (PP-AF). Using confocal microscopy and flow cytometry (FACS), we showed receptor-mediated and energy-dependent uptake of PP-AF by the hepatocarcinoma cell line HepG2 (Fig. 3).
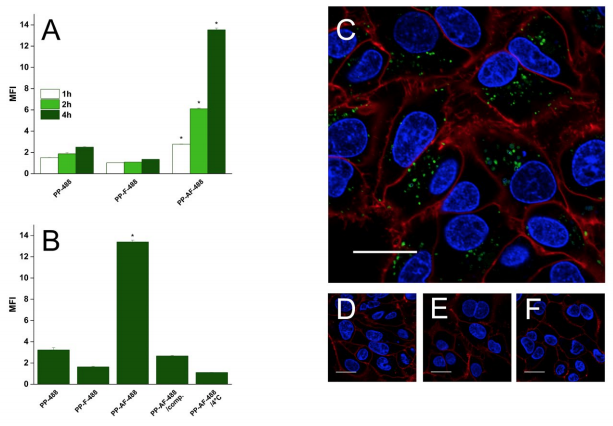
To confirm the biocompatibility of various PP formulations in vitro, we performed MTT assays. For all concentrations and formulations, cell viability was at least 80% (Fig. 4A). To investigate whether our polymersomes exhibit adverse effects in vivo, we used zebrafish embryos (ZFE). This vertebrate model is becoming increasingly recognized as an “intermediate” model for toxicity screening of small molecules but also nanoparticles before turning to experiments in rodents.4 ZFE with chorion as well as dechorionized ZFE were exposed up to 96 h postfertilization (hpf ) to the highest concentration of different PP formulations that were non-toxic in vitro (500 μg/mL). As a result, no signs of developmental toxicity were observed during the whole experiment (equal hatching rate, no malformations such as pericardial edema or tail malformations), suggesting good biocompatibility of all PP variants (Fig. 4B–D). In addition, preliminary experiments indicate that even direct injection of our polymersomes into the blood circulation of 72 hpf ZFE (using a microinjection device) did not result in acute toxicity. We conclude from these experiments that our polymersomes are well tolerated within a typical dose range suggested for use of nanomedicines.5
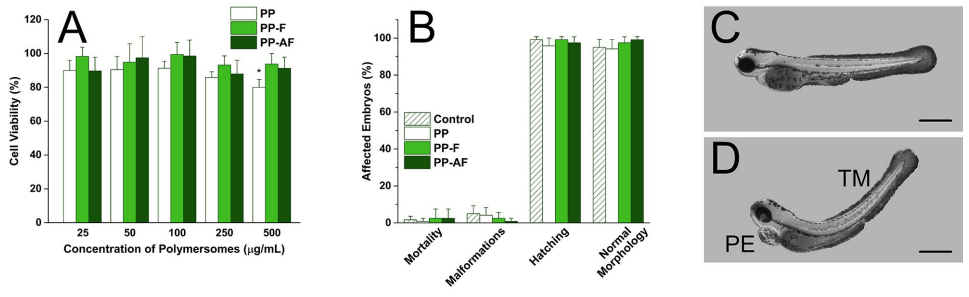
Conclusion
In conclusion, we achieved a targeted drug delivery system that is biocompatible in vitro and presumably also in vivo. It could be loaded with a hydrophilic model compound, and sustained release over a long time was achieved in DPBS as well as in serum protein containing media. Successful AF conjugation and therefore effective ASGPR targeting make our polymersomes a promising tool for clinical application in the field of liver disorders.
Acknowledgements
Adapted from Kiene et al.1 with permission from Elsevier. Financial support from NanoReg II, SCAHT, and Novartis University Basel Scholarship is acknowledged. In addition, we thank Prof. Affolter for providing ZFE and Dr. Chami and Mrs. Alampi (C-CINA) for cryo-TEM analysis.
References
1 Kiene, K, Schenk, SH, Porta, F, Ernst, A, Witzigmann, D, Grossen, P, Huwyler, J. PDMS-b-PMOXA polymersomes for hepatocellular targeting and assessment of toxicity. Eur. J. Pharm. Biopharm. 119: 322-332 (2017). doi:10.1016/j.ejpb.2017.07.002.
2 Lammers, T, Kiessling, F, Ashford, M, Hennink, W, Storm, G. Cancer nanomedicine: Is targeting our target ? Nat. Rev. Mater. 1: 1-4 (2016). doi:10.1038/natrevmats.2016.76.
3 Witzigmann, D, Quagliata, L, Schenk, SH, Quintavalle, C, Terracciano, LM, Huwyler, J. Variable asialoglycoprotein receptor 1 expression in liver disease: Implications for therapeutic intervention. Hepatol. Res. 46: 686-696 (2016). doi:10.1111/hepr.12599.
4 Rizzo, LY, Golombek, SK, Mertens, ME, Pan, Y, Laaf, D, Broda, J, Jayapaul, J, Möckel, D, Subr, V, Hennik, WE, Storm, G, Simon, U, Jahnen-Dechent, W, Kiessling, F, Lammers, T. In vivo nanotoxicity testing using the zebrafish embryo assay. J. Mater. Chem. B Mater. Biol. Med. 1: 3918-3925 (2013). doi:10.1039/C3TB20528B.
5 Kettiger, H, Sen, D, Schiesser, L, Rosenholm, JM, Huwyler, J. Comparative safety evaluation of silica-based particles. Toxicol. Vitr. 30: 355-363 (2015). doi:10.1016/j.tiv.2015.09.030.