Engineering Polymeric Nanocapsules with High Aspect Ratio as a High Drug-Payload and Long-circulating Drug Delivery System
Introduction
In recent years, research in nanotechnology for medical applications has focused on improving the efficiency of treatment and minimising side effects. Various strategies have been investigated to increase the time of circulation of nanoparticles in the body, provide more protection for the drug cargo to avoid pre-mature release of actives at non-specific sites, and to increase the efficiency of uptake. In this study, elongated polymeric nanocapsules were engineered as a high drug-payload and long circulating drug delivery system.
The relationship between the geometry of drug delivery nanomaterials and their cellular interactions has been widely investigated with the aim to decrease their internalisation by the macrophages in the immune system.1 Various non-spherical drug delivery nanoparticles, including cylindrical filomicelles2 and elliptical disk-shaped polystyrene particles3, have displayed a decreased accumulation in liver and stayed longer in systematic circulation. It is widely hypothesised that drug delivery systems with non-spherical geometry possess a greater chance to reach the site of action and thus improve the delivery efficiency.
Polymeric nanocapsules have attracted considerable attention in controlled drug delivery as their hollow interior provides a large void for encapsulation, while their thick polymer shell provides excellent stability, protection and slow release profiles.4 These benefits are especially pertinent in comparison to self-assembled polymeric architectures, like micelles and non-crosslinked vesicles, which can be prone to disassembly upon dilution in vivo, and undesirable premature release of cargo.
Polymeric nanocapsules are often prepared via templating methods with hard or soft spherical templates. However, using hard materials presents difficulties for removal of the template while soft templates are significantly less stable to provide a well-defined shape for the end products.5 An attractive alternative approach is the use of non-sacrificial liposome-type templates, such as dimethyldioctadecylammonium bromide (DODAB) vesicles6, sodium dodecylbenzenesulfonate (SDBS) vesicles7, and liposomes derived from egg phosphatidylcholine (egg-PC)8 onto which or from polymeric shells can be prepared.
Elongated liposomes can be prepared by nanocrystallisation of drug inside the liposomes9. Exploring the potential to use drug nanocrystal loaded liposomes as nanocapsule templates can simultaneously (1) pre-load pharmaceutical actives that are sensitive to hard templates or challenging to post-load after the formation of nanocapsule, (2) vary the aspect ratio of the final nanocapsules by modifying the lipid composition in the initial liposomal formulation12 and (3) eliminate the need for template removal.
In this study, elongated liposomes encapsulating ciprofloxacin drug nanocrystals10 were used as non-sacrificial templates to facilitate the preparation of elongated polymeric nanocapsules. Ciprofloxacin drug nanocrystals were formed by freeze-thawing drug-loaded liposomes, which was previously applied as a high-payload pulmonary inhalation vehicle to decrease the dosage frequency for patients with cystic fibrosis.11 After the formation of elongated liposomes, short-chain reversible addition−fragmentation chain-transfer (RAFT) oligomers were adsorbed onto the liposome surface. The ‘living’ nature of the RAFT oligomers was then exploited to mediate further chain-extension and formation of the nanocapsule polymeric shell.
The efficiency of RAFT oligomer adsorption onto the surface of elongated liposome templates and subsequent template stability were investigated. The effect of ethylene glycol diacrylate (EGDA) as the cross-linker, method of monomer addition and kinetics of polymerisation were explored in order to determine the optimal conditions to form elongated nanocapsules (Fig 1).
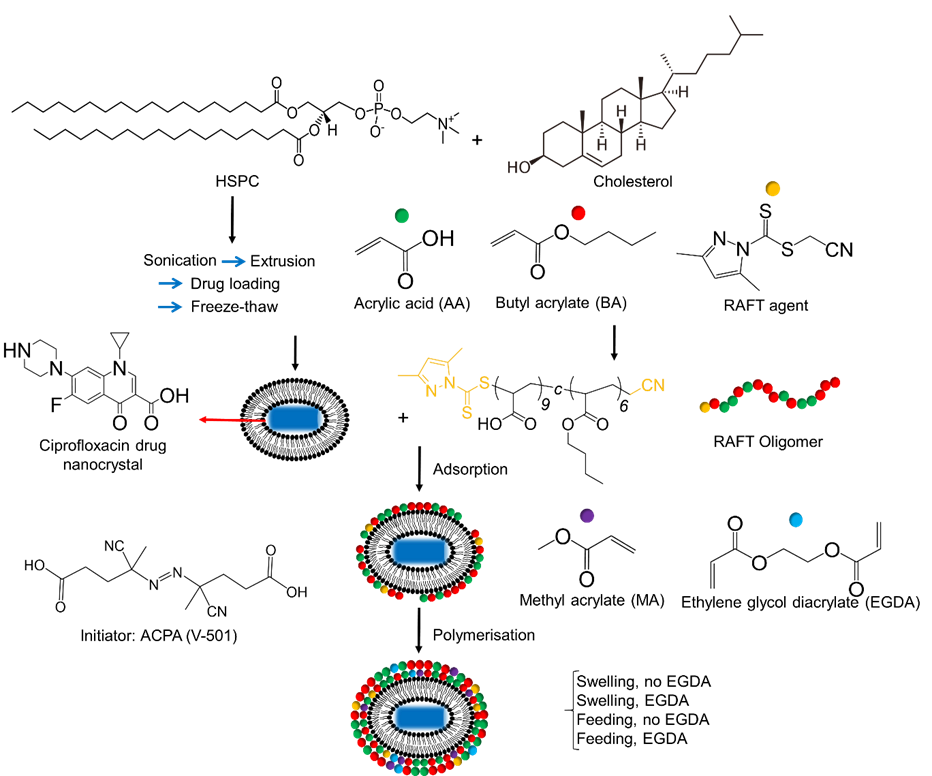
Elongated liposomes encapsulating drug nanocrystals
Thin film hydration method was used to prepare the lipid film, involving hydration with ammonium sulfate solution at pH 3 to set up the internal pH, sonication and extrusion through 200 nm membrane to decrease the size and polydispersity of the liposomes. Then ultrafiltration was performed to exchange the external buffer to PBS buffer at 7.4 to set up a pH gradient for active loading. Then the model drug, ciprofloxacin, was loaded at 70 °C and a 95% encapsulation efficiency was achieved. To obtain the drug nanocrystals and elongate the liposomes, a freeze-thaw method11 was used. The encapsulated liposomes were frozen in liquid nitrogen and thawed at room temperature. With the formation of ice crystals in the liposomes acting as the nuclei, ciprofloxacin crystallised and elongated the liposomes without breaking the lipid bilayer (Fig 2).
Cryo-TEM micrographs showed the successful formation of rod-shaped drug nanocrystals by freeze-thawing (Fig 3). All samples were vitrified but drug nanocrystals were only formed in the freeze-thawed samples; this indicates that the formation of drug nanocrystals only happened in the thawing process but not the freezing process in the freeze-thaw cycle. No nanocrystals were present in the extra-liposomal media indicating that drug nanocrystals only elongated the liposomes without breaking the lipid bilayer.10
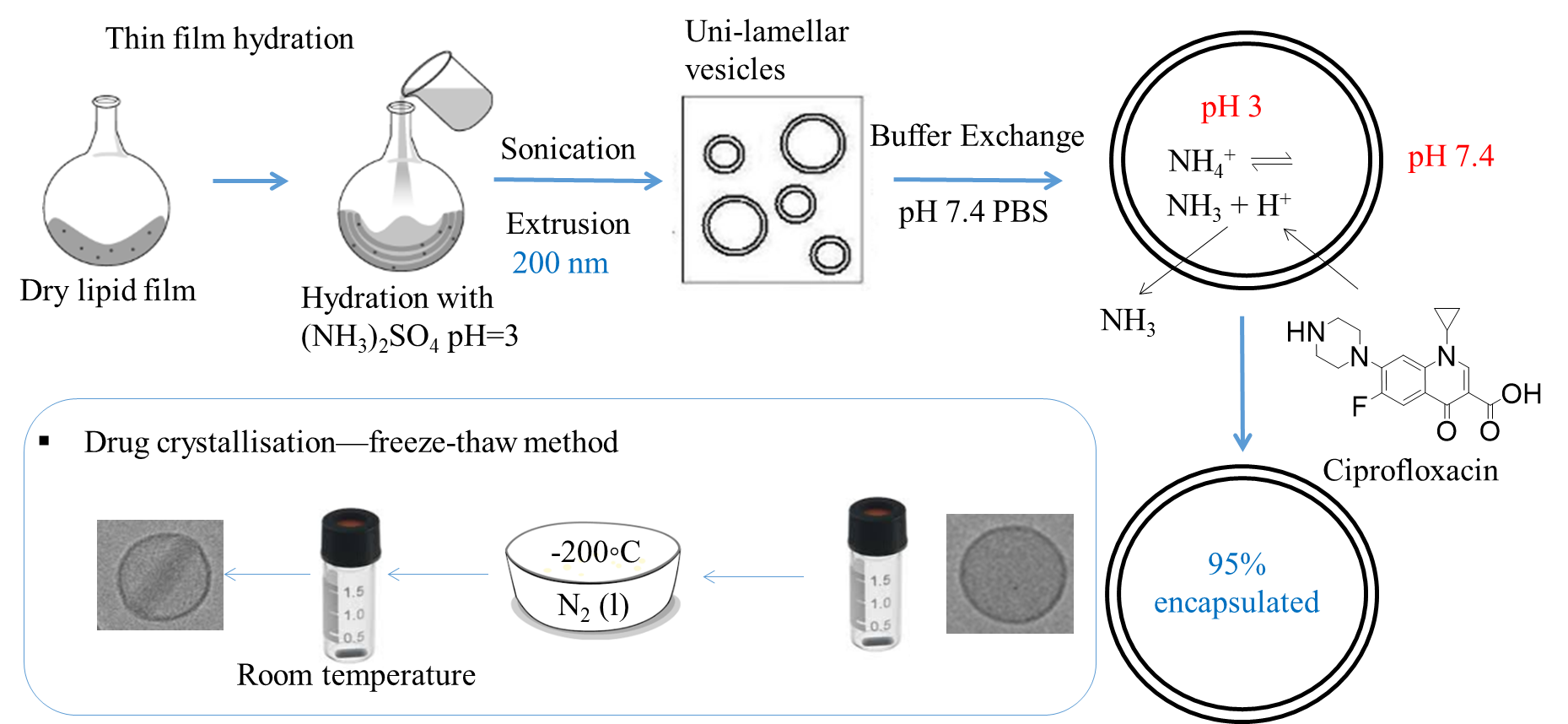
Growing a polymeric shell with the vesicle-templating method
Adsorption of RAFT oligomers to the liposomes was performed by adding equal volume of elongated liposomes to a solution of RAFT oligomers. The colloidal stability of the samples was maintained after adsorption (Fig 4) and no phase separation was observed, which was due to the balance of hydrophobicity provided by the butyl chain and hydrophilicity provided by the ionised carboxyl group in the RAFT oligomers, as this was first considered when the RAFT oligomer was designed.6 Ciprofloxacin nanocrystals also remained inside the liposomes, indicating that the adsorption process did not disrupt the lipid bilayer or the nanocrystals. With the liposomes containing nanocrystals, the elongated shape was also maintained after adsorption.
Polymerisations were performed under four different approaches to investigate the impact of two factors on the morphology of the nanocapsules; the use of a short-chain cross-linker, ethylene glycol diacrylate (EGDA), and the addition method of the monomers. Two addition methods were explored, including ‘swelling’, where monomers were added as a bolus to the reaction flask, and ‘feeding’, where monomers were added to the reaction flask at a speed of 2 mL/h with a syringe driver. Cryo-TEM micrographs (Fig 5) showed that nanocapsules with an empty interior space were formed, with much thicker shells compared to the thin bilayer of liposomes, confirming the adsorption process successfully driving the polymerisation reaction on the surface of the templates. Polymerised nanocapsules prepared without EGDA by swelling (Fig 5a) appeared to have inter-particle bonds between individual nanocapsules and the polymeric shell appeared to be wrinkly instead of smooth, possibly due to the lack of cross-linker and the swelling of the lipid bilayer. Nanocapsules prepared with EGDA by swelling (Fig 5b) eliminated the wrinkly shell but protrusion structures appeared at the ends of the capsules possibly due to the swelling of the monomers in the lipid bilayer causing phase separation between the formed polymer and liposome vesicles. Moving from swelling to feeding as the addition method, the protrusion structures disappeared in both experiments without (Fig 5c) and with EGDA (Fig 5d), indicating the successful set-up of RAFT equilibrium instead of the previous radical polymerisation. Various non-spherical morphologies were obtained but further improvements need to be performed to increase the population and consistency of elongated nanocapsules.
Conclusions
The study presented the successful formation of nanocapsules with non-spherical morphology by using liposomes encapsulating drug nanocrystals as the template. It presented a new opportunity for a high drug-payload, controlled release, and low dosage-frequency drug delivery system by combining the benefits of both liposome as a biocompatible drug carrier, polymer as an efficient protective material and elongated shape as a mechanism to decrease pre-systematic clearance. Optimisation leading to in vivo evaluation of pharmacokinetics and biodistribution are currently underway.
Acknowledgements
This research was funded by ARC Centre of Excellence in Convergent Bio-Nano Science & Technology (CBNS) through collaboration with Dr Alexander W. Jackson at the Institute of Chemical and Engineering Sciences (ICES), Agency for Science, Technology and Research (A*STAR), Singapore. We acknowledge the financial support from MITS, MGS and AINSE PGRA, cryo-TEM imaging facility and support from the Ramaciotti Centre for Cryo-Electron Microscopy and the Centre for BioImaging Sciences at the National University of Singapore.
References
1Champion, J. A.; Katare, Y. K.; Mitragotri, S. Particle shape: a new design parameter for micro-and nanoscale drug delivery carriers. J. Controlled Release 2007, 121 (1-2), 3-9.
2Geng, Y.; Dalhaimer, P.; Cai, S.; Tsai, R.; Tewari, M.; Minko, T.; Discher, D. E. Shape effects of filaments versus spherical particles in flow and drug delivery. Nat. Nanotechnol. 2007, 2 (4), 249.
3Champion, J. A.; Mitragotri, S. Role of target geometry in phagocytosis. Proc. Natl. Acad. Sci. U. S. A. 2006, 103 (13), 4930-4934.
4Meier, W. Polymer nanocapsules. Chem. Soc. Rev. 2000, 29 (5), 295-303.
5Wichaita, W.; Polpanich, D.; Tangboriboonrat, P. Review on synthesis of colloidal hollow particles and their applications. Ind. Eng. Chem. Res. 2019, 58 (46), 20880-20901.
6Rusli, W.; Jackson, A.; van Herk, A. A Roadmap towards Successful Nanocapsule Synthesis via Vesicle Templated RAFT-Based Emulsion Polymerisation. Polymers 2018, 10 (7), 774.
7Morgan, J. D.; Johnson, C. A.; Kaler, E. W. Polymerisation of equilibrium vesicles. Langmuir 1997, 13 (24), 6447-6451.
8Gomes, J. F. P. d. S.; Sonnen, A. F.-P.; Kronenberger, A.; Fritz, J.; Coelho, M. Á. N.; Fournier, D.; Fournier-Nöel, C.; Mauzac, M.; Winterhalter, M. Stable polymethacrylate nanocapsules from ultraviolet light-induced template radical polymerisation of unilamellar liposomes. Langmuir 2006, 22 (18), 7755-7759.
9Li, T., Cipolla, D., Rades, T., and Boyd, B. J. (2018) Drug nanocrystallisation within liposomes. J. Controlled Release.
10Xiao, Y., Liu, Q., Clulow, A. J., Li, T., Manohar, M., Gilbert, E. P., de Campo, L., Hawley, A., and Boyd, B. J. PEGylation and Surface Functionalization of Liposomes Containing Drug Nanocrystals for Cell-targeted Delivery. Colloids and Surfaces B: Biointerfaces 2019, 182, 110362.
11Cipolla, D., Wu, H., Salentinig, S., Boyd, B., Rades, T., Vanhecke, D., Petri-Fink, A., Rothin-Rutishauser, B., Eastman, S., Redelmeier, T., Gonda, I., and Chan, H.-K. Formation of drug nanocrystals under nanoconfinement afforded by liposomes. RSC Adv. 2016, 6, 6223-6233.
12Li, T., Clulow, A. J., Nowell, C. J., Hawley, A., Cipolla, D., Rades, T., and Boyd, B. J. Controlling the size and shape of liposomal ciprofloxacin nanocrystals by varying the lipid bilayer composition and drug to lipid ratio. J. Colloid Interface Sci. 2019, 555, 361-372.