Formulation and Delivery of Mono-N-Oxide Vinblastine Liposomal Formulation for Targeting Non-Small Cell Lung Cancer with Hypoxic Environments
Introduction
In non-small cell lung cancer (NSCLC), the level of oxygenation surrounding the cancerous cells is low compared with normal tissue owing to the rapid growth of the tumor cells. The decreased blood circulation results in development of pockets of lower oxygen levels, resulting in hypoxia.1 This abnormality in the tumor microenvironment results in a unique condition not prevalent in normal tissues. A number of functional groups such as N-oxides, nitroaromatics, and quinones can be reduced by several endogenous reductive enzymes that are overexpressed in the hypoxic tumor tissues.2 Forming a prodrug by incorporating these groups, which can undergo reduction to the active moiety in the hypoxic tissue, has been utilized recently as an approach for solid tumor targeting. Among the molecules developed is TH-302 a 2-nitroimidazole conjugated prodrug of bromo-isophosphoramide mustard, which is the only molecule that has progressed to the later stages of clinical development.3 However, the molecule did not meet its primary endpoint of improving overall survival. Following the same strategy, Cascade Prodrug Inc. developed vinblastine–N-oxide (CPD100, U.S. patent 20120107230), an N-oxide prodrug for vinblastine (VBL). VBL is a common anticancer molecule and forms an important role in many chemotherapeutic regimens, but like any other antitumor agent, it has severe side effects associated with its use, such as peripheral neuropathy and nervous system toxicity.4 The prodrug preferentially reduces at its nitrogen atom in cells or tissues by various endogenous reductases found only in hypoxic conditions, to the active moiety VBL, thus resulting in selective targeting (Figs. 1 and 2).
Preliminary data indicate that CPD100 has a plasma half-life (t1/2) of 0.44 h and a maximum tolerated dose (MTD) of 40 mg/ kg compared with VBL’s plasma t1/2 of 4.5 h and MTD of 5 mg/ kg in mice. Thus, although the prodrug displays an excellent safety and toxicity profile compared with the parent compound, its potential use is limited by its unfavorable pharmacokinetic profile, specifically the short t1/2. Thus, a drug delivery system is needed to increase the circulation half-life and efficacy of CPD100.
Liposomes are colloidal carriers that consist of an aqueous core enclosed by a concentric lipid bilayer vesicle capable of loading both hydrophilic and hydrophobic moieties. They usually range between 0.025 and 2.5 µm in diameter. A liposomal delivery system increases the efficacy of a drug by increasing the therapeutic concentration of the drug at the site of action and/or by decreasing the toxicity of the drug to normal tissues. The encapsulation of drugs in liposomes results in increasing the solubility and stability of drugs in vivo and has a major effect on the pharmacokinetics of drugs.5 In this work, we have developed a sphingomyelin/cholesterol liposomal formulation of CPD100 (CPD100Li), and we assessed the safety, efficacy, and pharmacokinetic profiles in in vitro and in vivo models.
Experimental Methods
Preparation and Characterization of Liposomes.
CPD100Li was prepared using a pH-gradient method utilizing sphingomyelin/cholesterol at 55:45 molar ratios. The lipidic thin film was formed by solvent evaporation and was rehydrated at 65°C for 30 min with 300 mM MgSO4 to form multilamellar vesicles (MLV). The resulting MLVs were extruded and purified using a Sephadex PD 10 column equilibrated with sucrose, 4-(2-hydroxyethyl)-1-piperazineethanesulfonic acid (HEPES), and ethylenediaminetetraacetic acid (EDTA) buffer (SHE) to generate the large unilamellar vesicles (LUV). CPD100 was loaded into the liposomes in the presence of A23187 ionophore via a pH gradient method.
CPD100Li was characterized using high-performance liquid chromatography (HPLC), cryo-transmission electron microscopy (Cryo-TEM), and dynamic light scattering (DLS).
In vitro Cell Proliferation Studies. The effects of empty liposomes (lipid concentration at 12.5 mM), CPD100Li (lipid concentration 0.5 mM to 2 nM), and CPD100 in dimethyl sulfoxide (DMSO) (lipid concentration 1 mM to 20 nM) were studied in adenocarcinomic human alveolar basal epithelial (A549) cells at two oxygen levels, normoxic (18%) and hypoxic (1.5%). Cell viability was assessed at 72 h using fluorescence measurements per the Cell Titer-Blue® protocol at 550 nm excitation and 590 nm emission. All the experiments were performed in quadruplicate.
In vivo Studies. The dose-limiting toxicity (DLT) and MTD were determined in nude athymic female mice by intravenous (i.v.) administration every 7 days for two cycles with no treatment for 3 weeks after the first cycle, as per the National Cancer Institute (NCI) Developmental Therapeutics Program protocol. Groups included empty liposomes (vehicle, lipid dose = 50 mg/kg), CPD100, and CPD100Li. CPD100Li efficacy was evaluated in an A549 tumor xenograft model with two cycles of dosing, and the percent changes in tumor volume and body weight were monitored for 90 days. The pharmacokinetic profile of CPD100Li was assessed in mice dosed at 30 mg/kg and fitted into a noncompartmental model to determine the clearance, volume of distribution, and circulation half-life.
Results and Discussion
Preparation and Characterization of Liposomes.
The CPD100 loading in the CPD100Li was 5.5 mg/mg, and the liposomes were stable for 48 h with a z-average diameter of 171 ± 3.44 nm and a polydispersity index (PDI) of 0.095 ± 0.038. Cryo-TEM of the CPD100Li showed the presence of more LUVs compared with MLVs, which were observed in the drug-free liposomes. There was no drug precipitation or electron-dense region in the CPD100Li, which was observed for other liposomal formulations as in CPT-11 and doxorubicin.7,8 This may be owing to the high hydrophilicity of CPD100, which prevented the precipitation.
In vitro Cell Proliferation Studies. The IC50 values (the concentration of an inhibitor at which the response is reduced by half ) of CPD100 in normoxia and hypoxia were 24,152 ± 2,185 and 11,600 ± 1,396 nM, respectively. In contrast, the IC50 values for CPD100Li in normoxia and hypoxia were 527 ± 99 and 132 ± 18 nM respectively. The empty liposomes at different lipid concentrations produced no antiproliferative effect in A549 cells under normoxic and hypoxic conditions compared with untreated cells. As expected, the data indicated a stronger antiproliferative effect for CPD100 under hypoxic conditions compared with normoxic conditions. The liposomal formulation for CPD100 was more potent than the free compound, possibly owing to a higher drug uptake by the cells due to liposomal encapsulation. Lipid nanocarriers tend to increase the penetration of chemotherapeutic agent inside the cells, and this combined with selective hypoxia activation of CPD100 made them more toxic to the cells.
In vivo Studies.The DLT for CPD100 and CPD100Li was 45 mg/kg. The MTD for CPD100 and CPD100Li was 40 mg/kg, indicating that the liposomal formulations did not change the acute toxicity profile of the CPD100. The effect of CPD100Li and CPD100 on the volume of the A549 xenograft model is depicted in Figure 3. The CPD100Li treatment group was significantly lower in the percent increase in the tumor volume of the mice after 90 days compared with other treatment groups (Fig. 3). The median survival of the control and vehicle groups was not statistically different. In contrast, the median survival of the CPD100Li-treated animals was 90 days, compared with the CPD100-treated group. The concentration versus time profile of the sum total of CPD100 in plasma after the administration of CPD100Li and CPD100 is depicted in Figure 4. The circulation time of CPD100Li increased by 13-fold compared with CPD100. This was also accompanied by an increase in the volume of distribution and decrease in clearance of the CPD100Li compared with CPD100, as seen in Table 1.
In conclusion, the results of our study indicate that the pharmacokinetic and efficacy profile of the first hypoxia-activated prodrug liposomal formulation was superior to that of CPD100 in a non-small cell lung cancer xenograft model. Our results represent a novel and efficacious therapeutic strategy utilizing hypoxia for treatment of lung cancer.
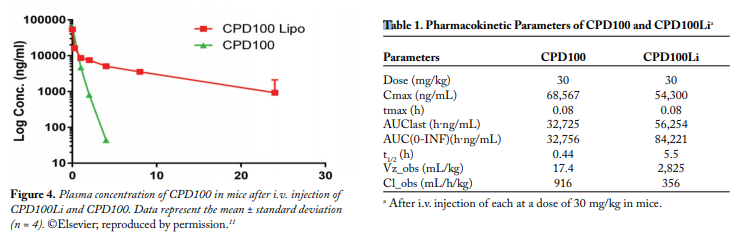
References 1. Zhang, J, Cao, J, Ma, S, Dong, R, Meng, W, Ying, M, Weng, Q, Chen, Z, Ma, J, Fang, Q, He, Q, Yang, B. Tumor hypoxia enhances non-small cell lung cancer metastasis by selectively promoting macrophage M2 polarization through the activation of ERK signaling. Oncotarget. 5(20): 9664-9677 (2014). 2. Brown, JM. Tumor hypoxia in cancer therapy. Methods Enzymol 435: 297-321 (2007). 3. Hansen, L. Threshold Pharmaceuticals announces its two phase 3 studies evaluating evofosfamide did not meet primary endpoints. http://investor.thresholdpharm.com/releasedetail.cfm?ReleaseID=945765 (2015). 4. Jordan, MA, Thrower, D, and Wilson, L. Mechanism of inhibition of cell proliferation by Vinca alkaloids. Cancer Res. 51(8): 2212-2222 (1991). 5. Drummond, DC, Meyer, O, Hong, K, Kirpotin, DB, Papahadjopoulos, D. Optimizing liposomes for delivery of chemotherapeutic agents to solid tumors. Pharmacol. Rev. 51(4): 691-743 (1999). 6. Fenske, DB, Wong, KF, Maurer, E, Maurer, N, Leenhouts, JM, Boman, N, Amankwa, L, Cullis, PR. Ionophore-mediated uptake of ciprofloxacin and vincristine into large unilamellar vesicles exhibiting transmembrane ion gradients. Biochim. Biophys. Acta 1414(1-2): 188-204 (1998). 7. Li, X, Hirsh, DJ, Cabral-Lilly, D, Zirkel, A, Gruner, SM, Janoff, AS, Perkins, WR. Doxorubicin physical state in solution and inside liposomes loaded via a pH gradient. Biochim. Biophys. Acta 1415(1): 23-40 (1998). 8. Taggar, AS, Alnajim, J, Anantha, M, Thomas, A, Webb, M, Ramsay, E, Bally, MB. Copper-topotecan complexation mediates drug accumulation into liposomes. J. Controlled Release 114(1): 78-88 (2006). 9. Narvekar, M, Xue, HY, Eoh, JY, Wong, HL. Nanocarrier for poorly water-soluble anticancer drugs—Barriers of translation and solutions. AAPS PharmSciTech 15(4): 822-833 (2014). 10. Zhou, J, Patel, TR, Sirianni, RW, Strohbehn, G, Zheng, MQ, Duong, N, Schafbauer, T, Huttner, AJ, Huang, Y, Carson, RE, Zhang, Y, Sullivan, DJ, Jr, Piepmeier, JM, Saltzman, WM. Highly penetrative, drug-loaded nanocarriers improve treatment of glioblastoma. Proc. Natl. Acad. Sci. U.S.A. 110(29): 11751-11756 (2013). 11. Shah, VM, Nguyen, DX, Alfatease, A, Bracha, S, Alani, AW. Characterization of pegylated and non-pegylated liposomal formulation for the delivery of hypoxia activated vinblastine-N-oxide for the treatment of solid tumors. J. Controled Release 253:37-45.