Modular Assembly of Immune Signals into Polyelectrolyte Multilayers to Promote Immunological Tolerance
Introduction
During autoimmune diseases, such as multiple sclerosis (MS), the body incorrectly recognizes and attacks “self ” tissues. Conventional clinical therapies for MS employ broad immunosuppression, which has provided important benefits for patients but is noncurative and can compromise healthy, protective immune function.1 In contrast, experimental therapies aim to reprogram how the body responds specifically to myelin—the self-molecule attacked in MS—while leaving the rest of the immune system intact. One approach toward this goal has been the coadministration of self-antigens and regulatory signals that could bias differentiating immune cells toward regulatory functions and phenotypes, instead of inflammatory populations.2–4 New studies in mouse models and human autoimmune disease reveal that excess signaling through inflammatory pathways, such as toll-like receptors (TLRs), contributes to disease.5–10 Thus, we hypothesized that codelivery of a regulatory TLR9 ligand, GpG,11–12 with myelin peptide could reduce TLR-driven inflammatory cues that expand self-reactive T cells, instead promoting regulatory T cells (TREGS) that control disease in a myelin-specific manner.
Biomaterials can enable codelivery, while offering other attractive features—tunable loading and cargo protection, for example—that support in vivo delivery of therapeutics.2–4 However, many biomaterials also exhibit intrinsic inflammatory features that could exacerbate autoimmune disease.13–15 Thus, we designed immune polyelectrolyte multilayers (iPEMs) as nanostructured assemblies that mimic attractive features of biomaterials but are composed entirely of immune signals. Because no other polymers, matrices, or carrier components are needed, iPEMs allow simple, modular assembly of peptide antigens and regulatory signals at very high cargo densities. As described in our recent report, self-antigens and GpG can be assembled into iPEMs using electrostatic interactions without compromising the selectivity of molecular interactions.16 Further, iPEMs promote tolerogenic phenotypes and function in mouse cells in vitro and in vivo, restrain disease in a mouse model of MS, and control inflammation in human MS patient samples.16 Below, we highlight some of these findings, as well as our ongoing work to incorporate controlled release capabilities into the iPEM platform.
Experimental Methods
iPEMs composed of myelin peptide fragments conjugated to tri-arginine to confer positive charge (MOG-R3), and either a regulatory TLR9 ligand, GpG, or an irrelevant control oligonucleotide (CTRL) were deposited on either planar (i.e., silicon or quartz) or colloidal (i.e., calcium carbonate microparticle templates) substrates in a layer-by-layer fashion. Layer-dependent growth of iPEMs was quantified using ellipsometry, UV/Vis spectrophotometry, and fluorescence microscopy. In some experiments, myelin peptide was replaced with a degradable poly(beta amino ester) to facilitate controlled release of immune cargo from iPEMs. In studies on colloidal substrates, sacrificial microparticle templates were removed after assembly by incubation in 0.1M ethylenediaminetetraacetic acid (EDTA), followed by a phosphate-buffered saline (PBS) wash before use in cell or animal studies.
For in vivo studies, mice were induced with a common model of MS, experimental autoimmune encephalomyelitis (EAE), and either left untreated or treated subcutaneously with iPEMs. Mice were monitored for clinical symptoms of disease (i.e., paralysis) and weight loss using protocols approved and overseen by the University of Maryland, College Park, Institutional Animal Care and Use Committee in compliance with local, state, and federal guidelines. At the indicated time points, cells from the spleens were isolated and analyzed for the frequency of TREGS and myelin-triggered secretion of inflammatory cytokines.
Human MS patient peripheral blood mononuclear cell (PBMC) samples were collected in conjunction with the Institutional Review Board-approved VALOMS study protocol with informed signed consent. VALOMS is an observational study that has been initiated
by the Department of Veterans Affairs Multiple Sclerosis Center of Excellence–East to examine factors associated with disease progression among U.S. military veterans with MS. Frozen PBMC samples selected randomly from the patient sample repository were thawed, washed, and incubated with tolerogenic or control iPEMs, or in media alone. Cultures were then analyzed for metabolic activity (MTT) and cytokine secretion via a Luminex multianalyte system.
aFischell Department of Bioengineering, University of Maryland, 8228 Paint Branch Drive, College Park, MD 20742, U.S.A.
bDepartment of Neurology, University of Maryland School of Medicine, 655 West Baltimore Street, Baltimore, MD 21201, U.S.A.
cMultiple Sclerosis Center of Excellence–East, U.S. Department of Veterans Affairs, 655 West Baltimore Street, Baltimore, MD 21201, U.S.A.
dDepartment of Microbiology and Immunology, University of Maryland Medical School, 685 West Baltimore Street, HSF-I Suite 380, Baltimore, MD 21201, U.S.A.
eU.S. Department of Veterans Affairs, 10 North Greene Street, Baltimore, MD 21201, U.S.A.
fMarlene and Stewart Greenebaum Cancer Center, 22 South Greene Street, Baltimore, MD 21201, U.S.A.
Results and Discussion
iPEM Assembly and Characterization. MOG-R3 and GpG were assembled in a layer-by-layer fashion to form iPEMs (Fig. 1A). Fluorescence microscopy confirmed colocalization of both cargos (Fig. 1B), while ellipsometry and spectrophotometry revealed an increase in iPEM thickness (Fig. 1C) and relative cargo loading (Fig. 1D), respectively, as a function of the number of bilayers deposited on planar substrates. To explore the potential for controlled release of immune cargos from iPEMs, in some studies, myelin peptide was replaced with a cationic, degradable polymer (Fig. 1E). Spectrophotometry revealed an increase in cargo loading during iPEM assembly (Fig. 1F), followed by a subsequent decrease in loading upon incubation of iPEMs in buffer (Fig. 1G). These results demonstrate the flexibility and modularity of this platform.
To facilitate cell and animal studies, MOG-R3/GpG iPEMs were next deposited on sacrificial calcium carbonate microparticles. Fluorescence microscopy images indicated an increase in cargo loading as a function of the number of layers, which was confirmed quantitatively via pixel intensity analysis (Fig. 2A). Next, the relative input of immune cargos
was varied, resulting in iPEMs with tunable relative loading of MOG-R3 and GpG (Fig. 2B). Following assembly of iPEMs, microparticle templates were dissolved, leaving hollow capsules composed entirely of either myelin and GpG, or myelin and an irrelevant control oligonucleotide
(Fig. 2C). Together, the data above demonstrate that iPEMs can be assembled in a programmable manner, motivating studies to investigate the tolerogenic potential.
iPEMs Restrain Inflammation, Promote TREGS, and Halt Disease in a Model of MS. To explore the impact of iPEMs on immune cell function and phenotype in vivo, mice were induced with EAE and either left untreated or administered iPEMs on days 5 and 10 post-induction (Fig. 3A). As expected, restimulation of cells from the spleens of induced, untreated mice with myelin peptide triggered high levels of inflammatory
interleukin 17 (IL-17) (Fig. 3B), interferon-g (IFN-g) (Fig. 3C), and interleukin 6 (IL-6) (Fig. 3D) secretion compared with cells pulsed with irrelevant peptide (i.e., OVA323-339). In contrast, restimulation of cells from iPEM-treated mice resulted in low levels of cytokine secretion, even during restimulation with myelin peptide, indicating a reduced inflammatory response
to self-antigen (Fig. 3B–D). In similar studies, cells from the spleen were analyzed immediately (i.e., without restimulation) for the presence of TREGS, defined as CD4+/CD25+Foxp3+ cells. iPEM treatment drove a significant increase in the frequency of TREGS (Fig. 3E–F). Finally, continued monitoring of mice treated as in Figure 3A revealed that induced, untreated mice exhibited significant EAE-induced paralysis (Fig. 3G) and weight loss (Fig. 3I). In contrast, iPEMs prevented disease in 100% of mice and inhibited disease-associated weight loss (Fig. 3G–I).
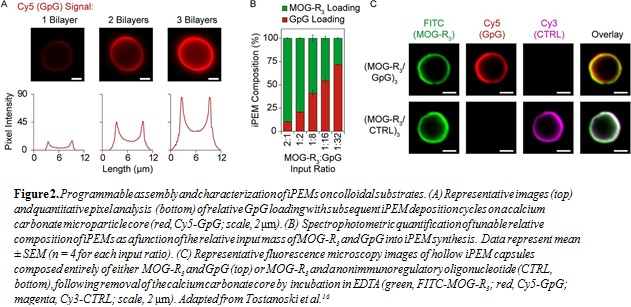
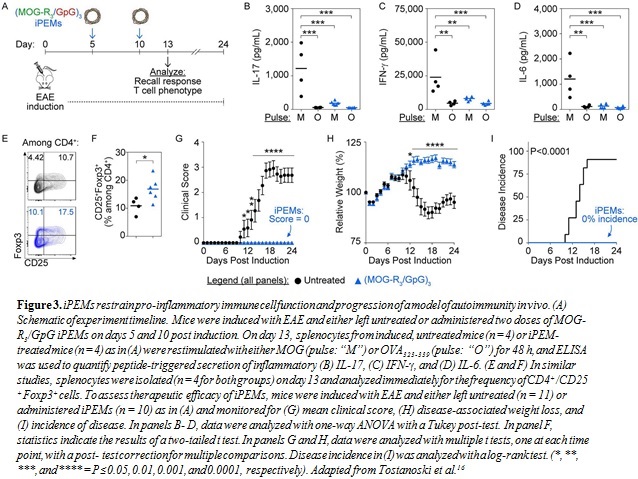
iPEMs Attenuate Inflammatory Response in Human MS Patient Samples. To investigate the translational potential of iPEMs to human disease, we drew on an approach used in recent clinical trials: ex vivo restimulation of PBMCs from MS patients.17 In our studies, we incubated PBMCs with either MOG-R3/GpG or MOG-R3/CTRL iPEMs to isolate any tolerogenic effects from inclusion of the TLR9 antagonist, GpG. MTT analysis revealed an increase in metabolic activity following treatment with either iPEM formulation (Fig. 4A). This result suggested the expected myelin-triggered increase in cell function associated with myelin- specific immune cells present during MS. However, despite similar levels of metabolic activity, GpG-containing iPEMs restrained inflammatory cytokine profiles compared with the control iPEM formulation. Excitingly, in nearly every case, necrosis factor
(TNF-a) (Fig. 4B), IL-6 (Fig. 4C), IL-10 (Fig. 4D), and IFN-y (Fig. 4E) levels were lower when PBMCs were treated with MOG-R3/GpG iPEMs compared with MOG-R3/CTRL iPEMs, although the specific cytokines for which these decreases were statistically significant varied across patients.
Conclusion
In our work, we have used three experimental systems—mouse cells, mouse models of MS, and human MS patient samples—to demonstrate that polyelectrolyte multilayer technology can be employed to promote immune tolerance. One of the unique opportunities with iPEMs and other tunable systems that permit controlled release is the ability to study how the kinetics of signal delivery impact the size or nature of immune response. Another ongoing direction is elucidation of whether late-stage treatment with iPEMs can reverse established disease in mice and drive remyelination in the central nervous system, critical criteria for experimental MS therapies. Further follow-up studies with statistically relevant sets of human patient samples could reveal the utility of iPEMs to combat human disease.
Acknowledgements
The authors thank L. Hester at the University of Maryland Cytokine Core Laboratory for her assistance with analysis of human PBMC samples and
K. Gaskell at the University of Maryland Department of Chemistry and Biochemistry for her assistance with atomic force microscopy analyses. The authors acknowledge the VALOMS investigators. This work was supported by the National Multiple Sclerosis Society award nos. RG-1501-02968 and PP2103, NSF CAREER award no. 1351688, the University of Maryland Venture Fund, the Maryland Innovation Initiative, and Veterans Affairs nos. I01BX007080 and RX000293. L.H.T. is a NSF Graduate Fellow no. DGE1322106. B.X. is a Howard Hughes Medical Institute Undergraduate Research Fellow supported by a grant to the University of Maryland from the Howard Hughes Medical Institute through the Science Education Program. Y.C. is a trainee on NIH Grant no. T32 CA154274. J.I.A. is a trainee on NIH Grant no. T32 AI089621 and a Graduate Fellow of the American Association of Pharmaceutical Scientists. C.M.J. is a Damon Runyon Rachleff Innovator supported by the Damon Runyon Foundation (no. DRR3415) and a Young Investigator of the Alliance for Cancer Gene Therapy (no. 15051543) and the Melanoma Research Alliance (no. 348963). This article draws heavily on our recent ACS Nano paper (16); figures are reproduced here in accordance with the CC-BY license.
References
- Cross, AH, Naismith, RT. Established and novel disease-modifying treatments in multiple sclerosis. J. Intern. Med. 275(4): 350-63 (2014).
- Northrup, L, Christopher, MA, Sullivan, BP, Berkland, C. Combining antigen and immunomodulators: Emerging trends in antigen-specific immunotherapy for autoimmunity. Adv. Drug Deliv. Rev. 98: 86-98 (2016).
- Maldonado, RA, LaMothe, RA, Ferrari, JD, Zhang, AH, Rossi, RJ Kolte, PN, Griset, AP, O’Neil, C, Altreuter, DH, Browning, E, Johnston, L, Farokhzad, OC, Langer, R, Scott, DW, von Andrian, UH, Kishimoto, TK. Polymeric synthetic nanoparticles for the induction of antigen-specific immunological tolerance. Proc. Natl. Acad. Sci. U.S.A. 112(2):E156- E165 (2015).
- Yeste, A, Nadeau, M, Burns, EJ, Weiner, HL, Quintana, FJ. Nanoparticle-mediated codelivery of myelin antigen and a tolerogenic small molecule suppresses experimental autoimmune encephalomyelitis. Proc. Natl. Acad. Sci. U.S.A. 109(28): 11270-5 (2012).
- Prinz, M, Garbe, F, Schmidt, H, Mildner, A, Gutcher, I, Wolter, K, Piesche, M, Schroers, R, Weiss, E, Kirschning, CJ, Rochford, CD, Bruck, W, Becher, B. Innate immunity mediated by TLR9 modulates pathogenicity in an animal model of multiple sclerosis. J. Clin. Invest. 116(2): 456-64 (2006).
- Balashov, KE, Aung, LL, Vaknin-Dembinsky, A, Dhib-Jalbut, S, Weiner, HL. Interferon-beta inhibits toll-like receptor 9 processing in multiple sclerosis. Ann. Neurol. 68(6): 899-906 (2010).
- Aung, LL, Fitzgerald-Bocarsly, P, Dhib-Jalbut, S, Balashov, K. Plasmacytoid dendritic cells in multiple sclerosis: Chemokine and chemokine receptor modulation by interferon-beta. J. Neuroimmunol. 226(1-2): 158-64 (2010).
- Derkow, K, Bauer, JM, Hecker, M, Paap, BK, Thamilarasan, M, Koczan, D, Schott, E, Deuschle, K, Bellmann-Strobl, J, Paul, F, Zettl, UK, Ruprecht, K, Lehnardt, S. Multiple sclerosis: Modulation of toll-like receptor (TLR) expression by interferon-beta includes upregulation of TLR7 in plasmacytoid dendritic cells. PLoS One 8(8):e70626 (2013).
- Kelly, PN, Romero, DL, Yang, Y, Shaffer, AL, Chaudhary, D, Robinson, S, Miao, W, Rui, L, Westlin, WF, Kapeller, R, Staudt, LM. Selective interleukin-1 receptor–associated kinase 4 inhibitors for the treatment of autoimmune disorders and lymphoid malignancy. J. Exp. Med. 212(13): 2189-2201 (2015).
- Reynolds, JM, Martinez, G., Chung, Y, Dong, C. Toll-like receptor 4 signaling in T cells promotes autoimmune inflammation. Proc. Natl. Acad. Sci. U.S.A. 109(32): 13064-13069 (2012).
- Ho, PP, Fontoura, P, Platten, M, Sobel, RA, DeVoss, JJ, Lee, LY, Kidd, BA, Tomooka, BH, Capers, J, Agrawal, A, Gupta, R, Zernik, J, Yee, MK, Lee, BJ, Garren, H, Robinson, WH, Steinman, L. A suppressive oligodeoxynucleotide enhances the efficacy of myelin cocktail/IL-4-tolerizing DNA vaccination and treats autoimmune disease. J. Immunol. 175(9): 6226-6234 (2005).
- Ho, PP, Fontoura, P, Ruiz, PJ, Steinman, L, Garren, H. An immunomodulatory GpG oligonucleotide for the treatment of autoimmunity via the innate and adaptive immune systems. J. Immunol. 171(9): 4920-4926 (2003).
- Andorko, JI, Hess, KL, Pineault, KG, Jewell, CM. Intrinsic immunogenicity of rapidly-degradable polymers evolves during degradation. Acta Biomater. 32: 24-34 (2016).
- Moyano, DF, Goldsmith, M, Solfiell, DJ, Landesman-Milo, D, Miranda, OR, Peer, D, Rotello, VM. Nanoparticle hydrophobicity dictates im- mune response. J. Am. Chem. Soc. 134(9): 3965-3967 (2012).
- Sharp, FA, Ruane, D, Claass, B, Creagh, E, Harris, J, Malyala, P, Singh, M, O’Hagan, DT, Petrilli, V, Tschopp, J, O’Neill, LA, Lavelle, EC. Uptake of particulate vaccine adjuvants by dendritic cells activates the NALP3 inflammasome. Proc. Natl. Acad. Sci. U.S.A. 106(3): 870-875 (2009).
- Tostanoski, LH, Chiu, YC, Andorko, JI, Guo, M, Zeng, X, Zhang, P, Royal, W, Jewell, CM. Design of polyelectrolyte multilayers to promote immunological tolerance. ACS Nano 10(10): 9334-9345 (2016).
- Lutterotti, A, Yousef, S, Sputtek, A, Sturner, KH, Stellmann, JP, Breiden, P, Reinhardt, S, Schulze, C, Bester, M, Heesen, C, Schippling, S, Miller, SD, Sospedra, M, Martin, R. Antigen-specific tolerance by autologous myelin peptide-coupled cells: A phase 1 trial in multiple sclerosis. Sci. Transl. Med. 5(188): 188ra75 (2013). n